Marco Hartl
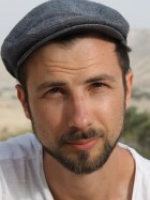
At BOKU University, Vienna, I specialized myself in Ecological Engineering and wrote a master thesis about an innovative two-stage vertical flow constructed wetland (CW) in the alpine region of Austria. Subsequently, I coordinated a research project at the world’s largest industrial CW, which treats oil produced water in the desert of Oman. In the super-W project, my CW experience will be combined with the exciting field of bio-electrochemical systems (BES).
The overall PhD’s objective is to provide sustainable wastewater treatment in rural areas by combining CWs with BES and recover valuable resources by doing so. CW are an economic and ecologic alternative for conventional wastewater treatment in small settlements and will be the main technology assessed in combination with BES - particularly Microbial Fuel Cells (MFC’s) and Microbial Electrolysis Cells (MEC’s). In MFC’s and MEC’s, active bacteria break down and remove organic matter from the wastewater while producing electricity. When incorporated in a CW, these BES can increase the treatment efficiency and thereby reduce the area required for purification. BES might also used as a biosensor, for example, to monitor the content of organic matter and other biologically active compounds throughout the CW system.
Status PhD
- PhD successfully defended
- Supervisors / promoters: Jaume Puigagut, Marianna Garfí (UPC Barcelona), Gijs Du Laing, Diederik Rousseau (UGent)
- Final title of the PhD thesis: Constructed wetlands operated as bioelectrochemical systems for improvement and control of wastewater treatment
- Place and date of PhD defense: Barcelona, February 2th 2020
- PhD degree awarding institutions: Universitat Politècnica de Catalunya; Ghent University
Publications arising from the PhD
- Hartl, M., Bedoya-Ríos, D. F., Fernández-Gatell, M., Rousseau, D. P., Du Laing, G., Garfí, M., & Puigagut, J. (2019). Contaminants removal and bacterial activity enhancement along the flow path of constructed wetland microbial fuel cells. Science of the total environment, 652, 1195-1208. https://doi.org/10.1016/j.scitotenv.2018.10.234
- Hartl, M., García-Galán, M. J., Matamoros, V., Fernández-Gatell, M., Rousseau, D. P., Du Laing, G., Garfí, M. & Puigagut, J. (2021). Constructed wetlands operated as bioelectrochemical systems for the removal of organic micropollutants. Chemosphere, 271, 129593. https://doi.org/10.1016/j.chemosphere.2021.129593
Other related publications (not included in the dissertation)
- Corbella, C., Hartl, M., Fernandez-gatell, M., & Puigagut, J. (2019). MFC-based biosensor for domestic wastewater COD assessment in constructed wetlands. Science of the total environment, 660, 218-226. https://doi.org/10.1016/j.scitotenv.2018.12.347
Link to PhD thesis (in digital repository)
http://hdl.handle.net/10803/669819
Short abstract/summary
The combination of constructed wetlands (CWs) and bioelectrochemical systems (BES) offers several opportunities. First of all, BES require a redox gradient between anode and cathode in order to drive bioelectrochemical processes, and CWs exhibit a pronounced natural redox gradient within the filter bed, especially when the CW is designed with a horizontal subsurface flow (HF) hydraulic regime. Electrochemically active bacteria (EAB) in BES utilize the energy gain from this redox gradient in order to act as catalysts and transfer electrons - derived from organic and inorganic matter oxidation - to the anode of the BES from where they flow to a higher redox potential at the cathode, creating a current in the opposite direction. Hence BES and CW-BES enable contaminant removal via a bioelectrochemical pathway while treating wastewater. If the BES are operated as microbial fuel cells (MFCs), a resistor or load is connected between anode and cathode, which additionally recovers a small amount of electric energy from the energy contained in the oxidized substrate. If the BES are operated as microbial electrolysis cells (MECs) an additional power source is applied instead of a load or resistance, hence no electricity is produced but otherwise thermodynamically unfavorable reactions can be achieved. Another advantage of MECs is that only an additional voltage of 0.2-0.8 V is required for water electrolysis to occur (usually 1.8-3.5 V are required), due to the current produced through the activity of EAB at the anode. Therefore, CWs operated as BES such as MFC (CW-MFC) and MEC (CW-MEC), profit from each other’s inherent features. The resulting synergies have the potential to improve contaminant removal and potentially allow for a broader range of contaminants to be treated. As a consequence, the relatively large required surface area per person equivalent (PE) of CWs could be reduced, which until now is one of the weak points of the technology. Furthermore, MFCs and CW-MFCs could potentially be used as a biosensor, since the produced current in an MFC is to some extent dependent and therefore correlated to the organic matter concentration in the wastewater. Hence, the main objective of this work was the improvement and control of wastewater treatment using CW-MFCs and CW-MECs. For this purpose, eight meso-scale CW-BES systems with 0.2 m2 Abstract X surface area were constructed, each with three BES in a row along the flow path, and liquid and gravel sampling ports in each transect in order to be able to observe processes within the filter beds. Unlike the majority of earlier research in the field, all systems received real urban wastewater and were operated in a realistic HF hydraulic regime. The first experiment investigated the application of CW-MFC systems as a biosensor for chemical oxygen demand (COD) at the influent of the systems. Triplicate meso-scale CW-MFCs were periodically fed with real urban wastewater and showed good bioindication responses between week 3 and 7 of operation (between an accumulated organic loading of ca. 100-200 g COD/m2 ). The majority of increases (75-80%) in COD concentrations at the influent could be detected after a response time of 2-4 h, however, the signal did not respond well to decreasing COD concentrations. Therefore, the CWMFCs were suggested to be used as an “alarm-tool” for sudden increases in COD or contamination events. Nevertheless, the application of the assessment tool would require more research concerning the biosensor design and operation, especially in regards to the prolongation of functionality with acceptable bioindication ranges, response times and sensitivity. The remaining experiments pivoted around improving contaminant removal using CW-BES. Initially duplicates of closedcircuit CW-MFC (CW-MFC+) were compared to an open-circuit CWMFC (CW-MFC-) control and a conventional CW-control over a period of 23 weeks. The CW-MFC+ fed with a continuous flow produced an extremely statistically significant higher current density than the CWMFC+ with intermittent flow, leading to the use of a continuous flow regime for the remaining experiments. Contaminant removal results showed no significant differences between tested organic loading rates (4.9±1.6, 6.7±1.4 and 13.6±3.2 g COD/m2 ·day), hydraulic regimes (intermittent vs. continuous flow) or different electrical connections. However, on average, CW-MFC+ with continuous flow outperformed other experimental conditions. In more detail, CW-MFC+ exhibited around 5% and 22% higher COD and ammonium removal, respectively, compared to conventional CW-control systems. Correspondingly, overall bacteria activity, as measured by the fluorescein diacetate technique, in CW-MFC+ was higher by 4% to 34% when compared to CW-control systems. Abstract XI For the next experiment, a duplicate of CW-MEC systems was added to the CW-MFC+, CW-MFC- and CW-control duplicates, and wastewater treatment performance was assessed again. Results showed that average ammonium and COD removal was higher in CWMEC (by 18% and 9%, respectively) and CW-MFC+ (by 16% and 6%, respectively) when compared to CW-control, while CW-MFCperformed similarly to the CW-control. This time also a microbial community analysis was performed and showed statistically significant differences in microbial structural composition of CW-MEC anodes and cathodes when compared to all other treatments. The most abundant species was Sphingobium yanoikuyae which has not been reported in CW-MEC, or in general in BES such as MFC or MEC before. However, the closely related genera Sphingomonas and Sphingopyxis were reported in other CW-MEC system. Probably due to the sampling method at the anode, only cathode samples of CW-MFC+ showed a microbial community significantly different from CW-MFC- and CWcontrol with relative high abundance of the species Lysinibacillus boronitolerans, which is closely related to Lysinibacillus sphaericus, a species which was also found in other MFC systems and was even identified to be electrochemically active. During the final investigation CW-MEC, CW-MFC+ and CWcontrol duplicates were fed with wastewater spiked with organic micropollutants, more precisely the four pharmaceuticals carbamazepine (CBZ), diclofenac (DCF), ibuprofen (IBU) and naproxen (NPX). Higher removal rates were obtained for three out of the four compounds (CBZ, DCF and NPX) with an increase of 10-17% in CWMEC and 5% in CW-MFC systems, compared to the CW-control. However, no statistically significant differences were found. IBU removal was similar amongst treatments. Taken all together, the initial experiment on the use of CWMFCs as a COD assessment tool revealed interesting results but more research in terms of strategies in order to maintain functional stability, as well as a reasonable response time, detection range and sensitivity are suggested in order to advance the technology. In terms of contaminant removal, CW-BES systems showed promising results especially for ammonium and certain organic micropollutants which are recalcitrant and not easily treated with other technologies. In any case, it is suggested to continue research and identify the precise processes, conditions and microbial communities which are responsible for these Abstract XII improvements, in order to be able to design tailor-made CW-BES systems for the respective treatment goals in the future.